Enzymes are protein molecules that can be defined as biological catalysts. A catalyst is, “A molecule which speeds up a chemical reaction but remains unchanged at the end of the reaction“.Virtually every metabolic reaction which takes place within a living organism is catalyzed by an enzyme and enzymes are therefore essential for life to exist. Many enzyme names end in –ase – for example Amylase and ATPase.
Intracellular and extracellular enzymes
Not all enzymes operate within cells. Those that do are described as intracellular. Enzymes that are secreted by cells and catalyze reactions outside cells are described as extracellular. Digestive enzymes in the gut are an example. Some organisms secrete enzymes outside their bodies. “Fungi” for example, often do this in order to digest the substrate on which they are growing.
Lock and Key and Induced Fit Hypotheses
Enzymes are “globular proteins”. Like all globular proteins, enzyme molecules are coiled into a precise three-dimensional shape, with hydrophilic R groups (sidechains) on the outside of the molecule ensuring that they are soluble. Enzyme molecules also have a special feature in that they possess an “active site” (Figure A). “The active site of an enzyme is a region, usually a cleft or depression, to which another molecule or molecules can bind”. This molecule is the substrate of the enzyme. The shape of the active site allows the substrate to fit perfectly. The idea that the enzyme has a particular shape into which the substrate fits exactly is known as “The Lock And Key Hypothesis“. The substrate is the key whose shape fits the lock of the enzyme. The substrate is held in place by temporary bonds that form between the substrate and some of the R groups of the enzyme’s amino acids. This combined structure is termed the enzyme-substrate complex.
Best safe and secure cloud storage with password protection
Get Envato Elements, Prime Video, Hotstar and Netflix For Free
Best Money Earning Website 100$ Day
#1 Top ranking article submission website
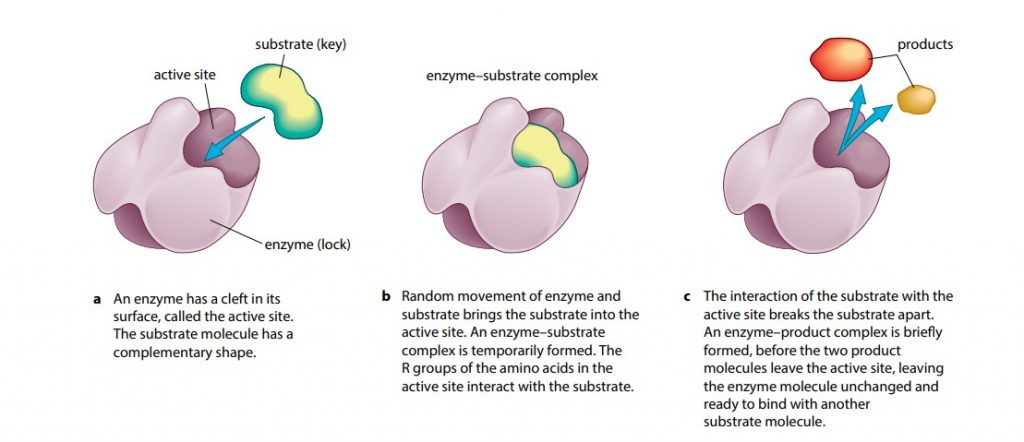
“Each type of enzyme will usually act on only one type of substrate molecule”. This is because the shape of the active site will only allow one shape of molecule to fit. The enzyme is said to be specific for this substrate. In 1959 the lock and key hypothesis was modified in the light of evidence that enzyme molecules are more flexible than is suggested by a-“rigid lock and key”. The modern hypothesis for enzyme action is known as “The Induced Fit Hypothesis”. It is basically the same as the lock and key hypothesis but adds the idea that the enzyme, and sometimes the substrate, can change shape slightly as the substrate molecule enters the enzyme, in order to ensure a perfect fit. This makes the catalysis even more efficient.
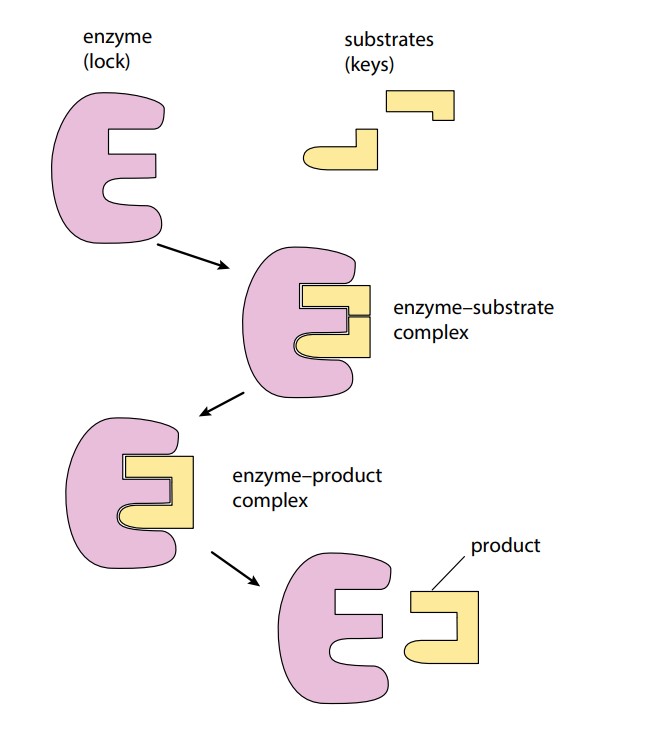
An enzyme may catalyze a reaction in which the substrate molecule is split into two or more molecules, as shown in Figure (A). Alternatively, it may catalyze the joining together of two molecules, as when making a dipeptide. A simplified diagram is shown in Figure (B). This diagram also shows the enzyme–product complex which is briefly formed before the release of the product. Interaction between the R groups of the enzyme and the atoms of the substrate can break, or encourage the formation of, bonds in the substrate molecule, forming one, two, or more products.
When the reaction is complete, the product or products leave the active site. The enzyme is unchanged by this process, so it is now available to receive another substrate molecule. The rate at which substrate molecules can bind to the enzyme’s active site, be formed into products, and leave can be very rapid.
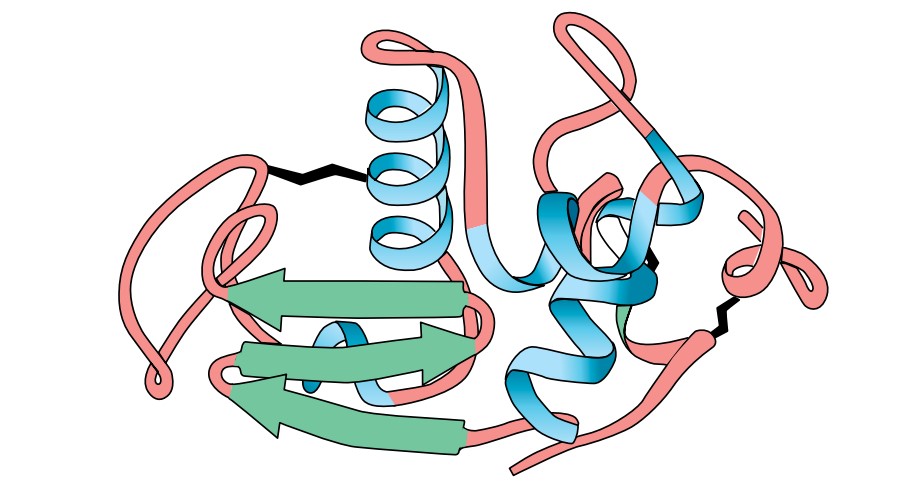
The interaction between the substrate and the active site, including the slight change in the shape of the enzyme (induced fit) which results from the binding of the substrate, is clearly shown by the enzyme lysozyme. Lysozyme is a natural defense against bacteria that is found in tears, saliva, and other secretions. It breaks the “polysaccharide” chains that form the cell walls of bacteria. The tertiary structure of the enzyme has already been shown in Figure (C). Figure (D) shows how part of the polysaccharide substrate is broken down in the active site.
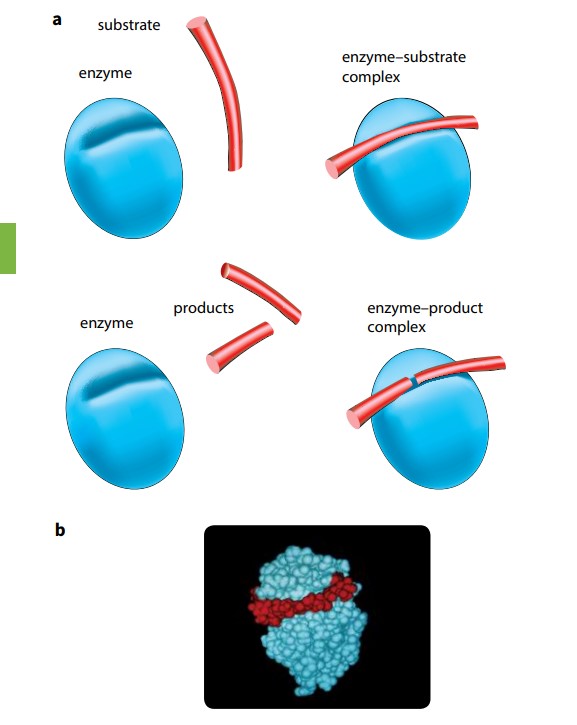
Enzymes reduce the activation energy
As catalysts, enzymes increase the rate at which chemical reactions occur. Most of the reactions which occur in living cells would occur so slowly without enzymes that they would virtually not happen at all. In many chemical reactions, the substrate will not be converted to a product unless it is temporarily given some extra energy. This energy is called activation energy (Figure Ex).
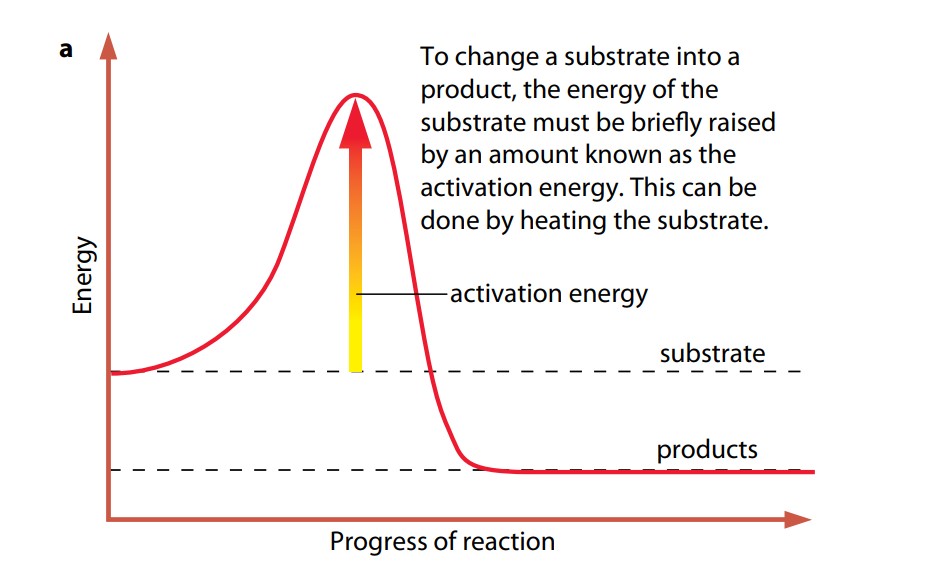
One way of increasing the rate of many chemical reactions is to increase the energy of the reactants by heating them. You have probably done this by heating substances in which you want to react together. In Benedict’s test for reducing sugar, for example, you need to heat Benedict’s reagent and sugar solution together before they will react.
Mammals such as humans also use this method of speeding up their metabolic reactions. Our body temperature is maintained at 37 °C, which is usually much warmer than the temperature of the air around us. But even raising the temperature of cells to 37 °C is not enough to give most substrates the activation energy which they need to change into products. Enzymes avoid this problem because they decrease the activation energy of the reaction which they catalyze. They do this by holding the substrate or substrates in such a way that their molecules can react more easily. Reactions catalyzed by enzymes will take place rapidly at a much lower temperature than they otherwise would.
Temperature and enzyme activity
Figure (E) shows how the rate of a typical “enzyme-catalyzed” reaction varies with temperature. At low temperatures, the reaction takes place only very slowly. This is because molecules are moving relatively slowly. Substrate molecules will not often collide with the active site, and so binding between substrate and enzyme is a rare event. As temperature rises, the enzyme and substrate molecules move faster. Collisions happen more frequently, so that substrate molecules enter the active site more often. Moreover, when they do collide, they do so with more energy. This makes it easier for bonds to be formed or broken so that the reaction can occur.
As the temperature continues to increase, the speed of movement of the substrate and enzyme molecules also continues to increase. However, above a certain temperature, the structure of the enzyme molecule vibrates so energetically that some of the bonds holding the enzyme molecule in its precise shape begin to break. This is especially true of hydrogen bonds. The enzyme molecule begins to lose its shape and activity and is said to be denatured. This is often irreversible. At first, the substrate molecule fits less well into the active site of the enzyme, so the rate of the reaction begins to slow down. Eventually, the substrate no longer fits at all, or can no longer be held in the correct position for the reaction to occur.
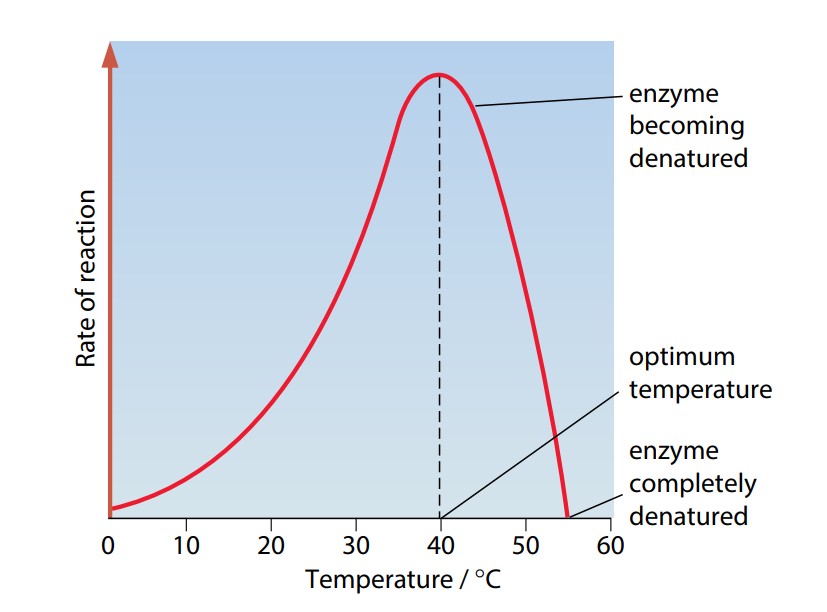
The temperature at which an enzyme catalyzes a reaction at the maximum rate is called the optimum temperature. Most human enzymes have an optimum temperature of around 40 °C. By keeping our body temperatures at about 37 °C, we ensure that enzyme-catalyzed reactions occur at close to their maximum rate. It would be dangerous to maintain a body temperature of 40 °C, as even a slight rise above this would begin to denature enzymes.
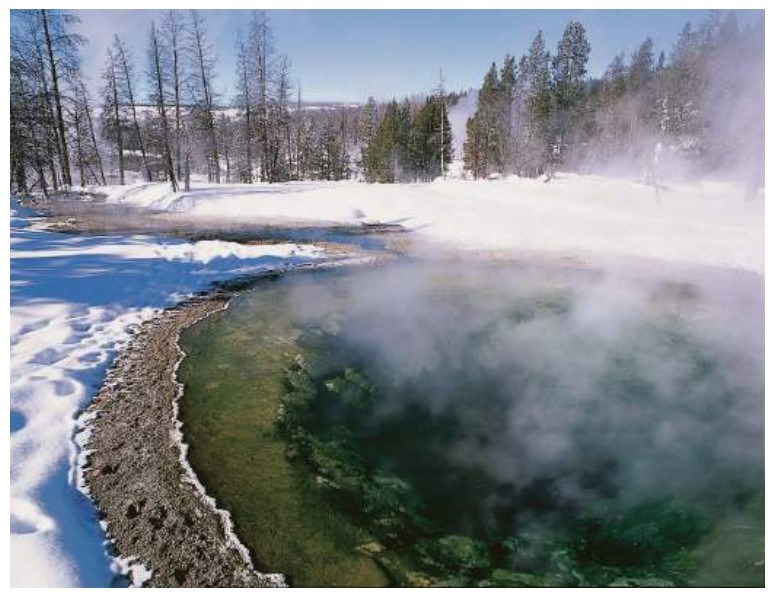
Enzymes from other organisms may have different optimum temperatures. Some enzymes, such as those found in bacteria that live in hot springs (Figure H), have much higher optimum temperatures. Some plant enzymes have lower optimum temperatures, depending on their habitat.
pH and enzyme activity
Figure (I) shows how the activity of an enzyme is affected by pH. Most enzymes work fastest at a pH of somewhere around 7–that is, in fairly neutral conditions. Some, however, such as the protease pepsin, which is found in the acidic conditions of the stomach, have a different optimum pH.
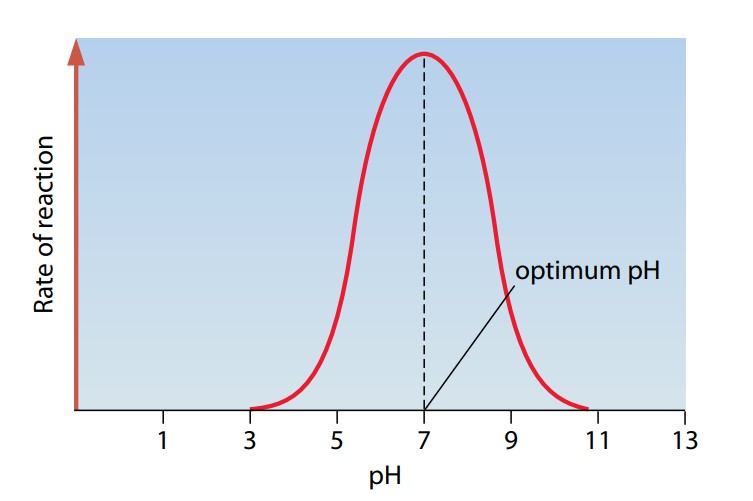
pH is a measure of the concentration of hydrogen ions in a solution. The lower the pH, the higher the hydrogen ion concentration. Hydrogen ions can interact with the R groups of amino acids – for example, by affecting ionization (the negative or positive charges) of the groups. This affects the ionic bonding between the groups which in turn affects the three-dimensional arrangement of the enzyme molecule. The shape of the active site may change and therefore reduce the chances of the substrate molecule fitting into it. A pH that is very different from the optimum pH can cause denaturation of an enzyme.
The course of a reaction
An investigation carries out into the rate at which substrate is converted into a product during an enzyme-controlled reaction. Figure (J) shows the results of such an investigation using the enzyme catalase. This enzyme is found in the tissues of most living things and catalyzes the breakdown of hydrogen peroxide into water and oxygen. (Hydrogen peroxide is a toxic product of several different metabolic reactions, and so it must be got rid of quickly.) It is an easy reaction to follow, as the oxygen that is released can be collected and measured.
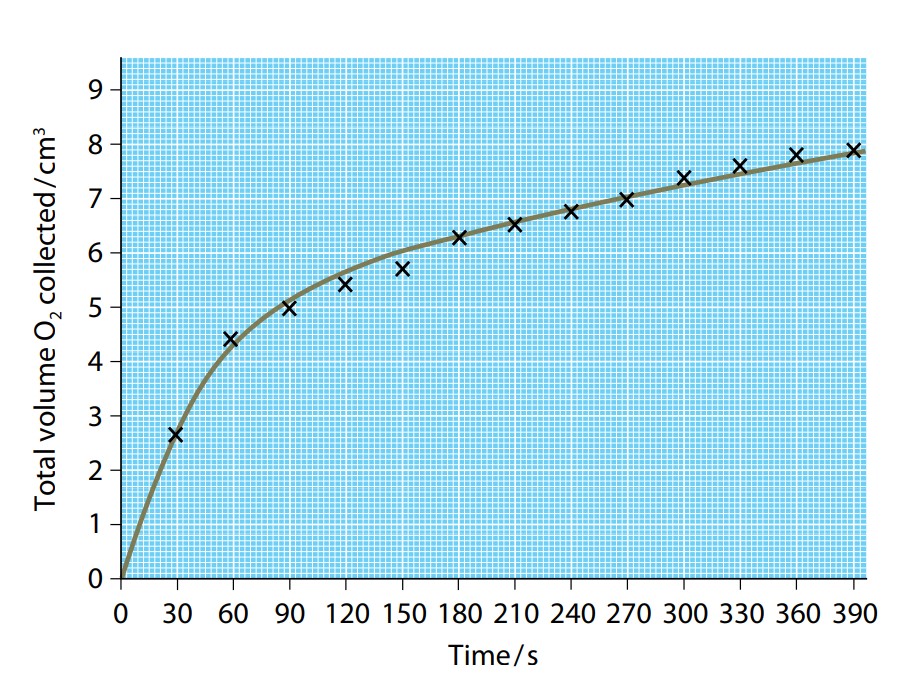
The explanation for the course of the reaction is quite straightforward. When the enzyme and substrate are first mixed, there are a large number of substrate molecules. At any moment, virtually every enzyme molecule has a substrate molecule in its active site. The rate at which the reaction occurs depends only on how many enzyme molecules there are and the speed at which the enzyme can convert the substrate into a product, release it, and then bind with another substrate molecule. However, as more and more substrate is converted into a product, there are fewer and fewer substrate molecules to bind with enzymes. Enzyme molecules may be ‘waiting’ for substrate molecules to hit their active sites. As fewer substrate molecules are left, the reaction gets slower and slower, until it eventually stops.
Enzyme Inhibitors
1.Competitive And Reversible Inhibition
As we have seen, the active site of an enzyme fits one particular substrate perfectly. It is possible, however, for some other molecule to bind to an enzyme’s active site if it is very similar in shape to the enzyme’s substrate. This would then inhibit the enzyme’s function. If an inhibitor molecule binds only briefly to the site, there is a competition between it and the substrate for the site. If there is much more of the substrate present than the inhibitor, substrate molecules can easily bind to the active site in the usual way, and so the enzyme’s function is unaffected. However, if the concentration of the inhibitor rises or that of the substrate falls, it becomes less and less likely that the substrate will collide with an empty site. The enzyme’s function is then inhibited. This is therefore known as competitive inhibition (Figure Ka). It is said to be reversible (not permanent) because it can be reversed by increasing the concentration of the substrate.
An example of competitive inhibition occurs in the treatment of a person who has drunk ethylene glycol. Ethylene glycol is used as an antifreeze and is sometimes drunk accidentally. Ethylene glycol is rapidly converted in the body to oxalic acid, which can cause irreversible kidney damage. However, the active site of the enzyme which converts ethylene glycol to oxalic acid will also accept ethanol. If the poisoned person is given a large dose of ethanol, the ethanol acts as a competitive inhibitor, slowing down the action of the enzyme on ethylene glycol for long enough to allow the ethylene glycol to be excreted.
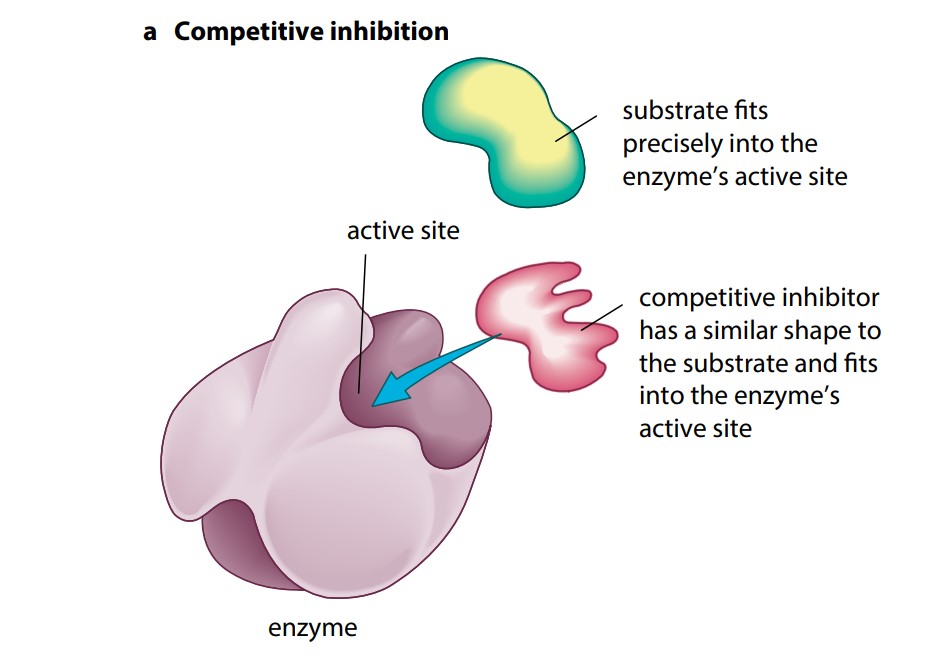
2.Non-competitive, reversible inhibition
A different kind of reversible inhibition takes place if a molecule can bind to another part of the enzyme rather than the active site. While the inhibitor is bound to the enzyme it can seriously disrupt the normal arrangement of hydrogen bonds and hydrophobic interactions holding the enzyme molecule in its three-dimensional shape. The resulting distortion ripples across the molecule to the active site, making the enzyme unsuitable for the substrate. While the inhibitor is attached to the enzyme, the enzyme’s function is blocked no matter how much substrate is present, so this is an example of noncompetitive inhibition (Figure Kb).
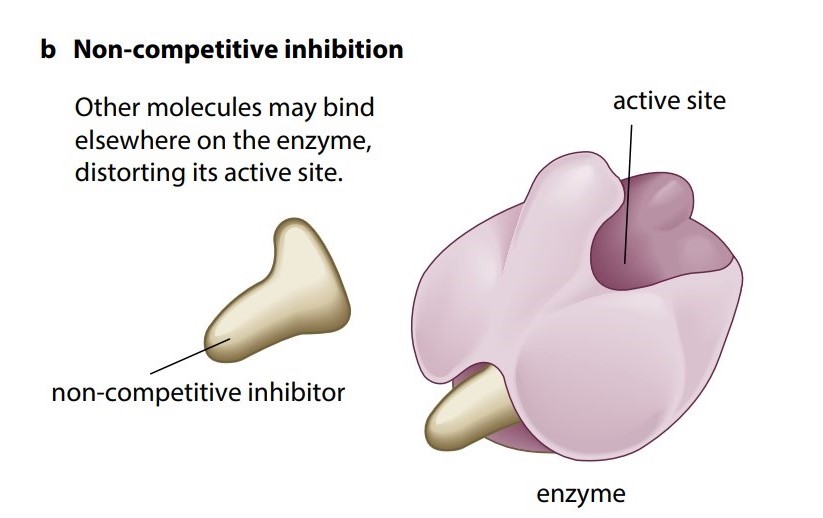
However inhibition of enzyme function can be lethal, but in many situations inhibition is essential. For example, metabolic reactions must be very finely controlled and balanced, so no single enzyme can be allowed to ‘run wild’, constantly churning out more and more products.
Basic Concept Of The Variation Of Enzyme
There is enormous variation in the speed at which different enzymes work. A typical enzyme molecule can convert around one thousand substrate molecules into a product per second. This is known as the turnover rate. The enzyme carbonic anhydrase is one of the fastest enzymes known. It can remove 600 000 molecules of carbon dioxide from respiring tissue per second, roughly 107 times as fast as the reaction would occur in the absence of the enzyme. It has presumably evolved such efficiency because a build-up of carbon dioxide in tissues would quickly become lethal. Speeds such as these are only possible because molecules within cells move about very quickly by diffusion over short distances, with tens or hundreds of thousands of collisions per second occurring between enzyme and substrate molecules.
Immobilizing enzymes
Enzymes have an enormous range of commercial applications – for example, in medicine, food technology, and industrial processing. Enzymes are expensive. No company wants to have to keep buying them over and over again if it can recycle them in some way. One of the best ways of keeping costs down is to use immobilized enzymes.
enzyme immobilization has several obvious advantages compared with just mixing up the enzyme with its substrate. If we just mixed lactase with milk, we would have a very difficult task to get the lactase back again. Not only would we lose the lactase, but also we would have milk contaminated with the enzyme. Using immobilized enzymes means that we can keep and re-use the enzymes and that the product is enzyme-free.
Another advantage of this process is that the immobilized enzymes are more tolerant of temperature changes and pH changes than enzymes in solution. This may be partly because their molecules are held firmly in shape by the alginate in which they are embedded, and so do not denature as easily. It may also be because the parts of the molecules that are embedded in the beads are not
fully exposed to the temperature or pH changes.
Summary:
Enzymes are globular proteins that catalyze metabolic reactions. Each enzyme has an active site with a flexible structure that can change shape slightly to fit precisely the substrate molecule. This is called the induced fit hypothesis. When the substrate enters the active site, an enzyme-substrate complex is temporarily formed in which the R groups of the amino acids in the enzyme hold the substrate in place.
Enzymes may be involved in reactions that break down molecules or join molecules together. They work by lowering the activation energy of the reactions they catalyze.
Various factors affect the rate of activity of enzymes. Four important factors are enzyme concentration:
- Substrate
- Concentration
- Temperature
- pH
“The greater the concentration of the enzyme, the faster the rate of reaction“, provided there are enough substrate molecules present. During enzyme reactions, rates slow down as substrate molecules are used up.
- Each enzyme has an optimum temperature at which it works fastest. As temperature increases above the optimum temperature, the enzyme gradually denatures (loses its precise tertiary structure). When an enzyme is completely denatured, it ceases to function, but denaturation is sometimes reversible.
- Each enzyme has an optimum pH. Some enzymes operate within a narrow pH range; some have a broad pH range.
Thank you for your sharing. I am worried that I lack creative ideas. It is your article that makes me full of hope. Thank you. But, I have a question, can you help me? https://www.binance.info/zh-CN/join?ref=FIHEGIZ8