Globular and fibrous proteins
A protein whose molecules curl up into a ‘ball’ shape, such as myoglobin or hemoglobin, is known as a globular protein. In a living organism, proteins may be found in cells and in other aqueous environments such as blood, tissue fluid and in phloem of plants. Globular proteins usually curl up so that their non-polar, hydrophobic R groups point into the center of the molecule, away from
their watery surroundings. Water molecules are excluded from the center of the folded protein molecule. The polar, hydrophilic R groups remain on the outside of the molecule. Globular proteins, therefore, are usually soluble, because water molecules cluster around their outward pointing hydrophilic R groups (Figure J).
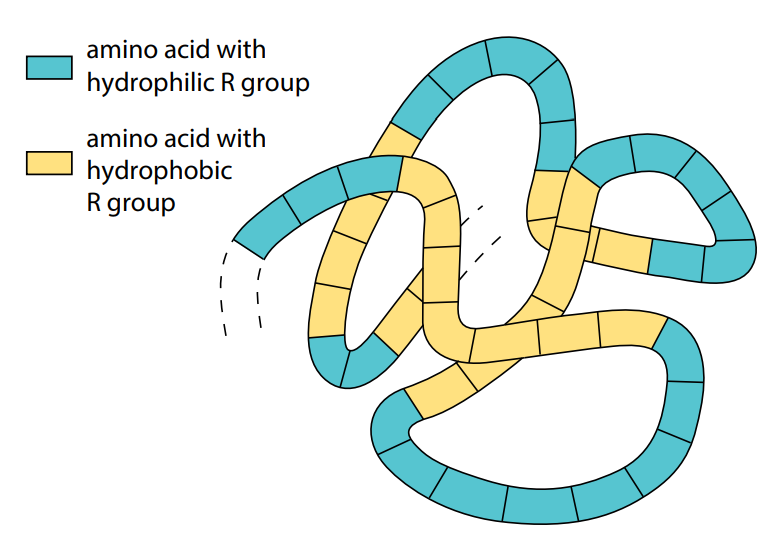
Many globular proteins have roles in metabolic reactions. Their precise shape is the key to their functioning. Enzymes, for example, are globular proteins. Many other protein molecules do not curl up into a ball, but form long strands. These are known as fibrous proteins. Fibrous proteins are not usually soluble in water and most have structural roles. For example, keratin
forms hair, nails and the outer layers of skin, making these structures waterproof. Another example of a fibrous protein is collagen.
Hemoglobin – a globular protein
Hemoglobin is the oxygen-carrying pigment found in red blood cells, and is a globular protein. We have seen that it is made up of four polypeptide chains, so it has a quaternary structure. Each chain is itself a protein known as globin. Globin is related to myoglobin and so has a very similar tertiary structure (Figures K and L). There are many types of globin – two types are used to make hemoglobin, and these are known as alpha-globin (α-globin) and beta-globin (β-globin). Two of the hemoglobin chains, called α chains, are made from α-globin, and the other two chains, called β-chains, are made from β-globin.
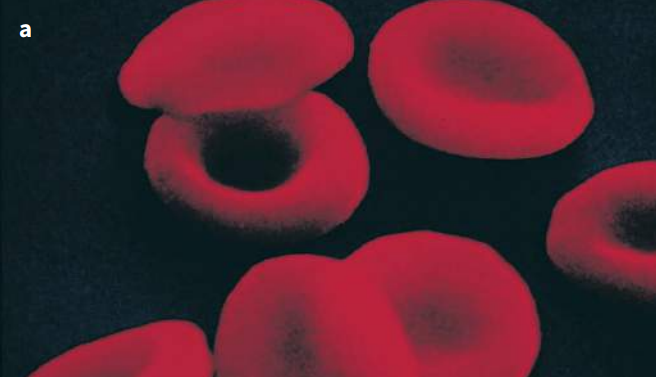
The hemoglobin molecule is nearly spherical (Figure K). The four polypeptide chains pack closely together, their hydrophobic R groups pointing in towards the center of the molecule, and their hydrophilic ones pointing outwards.
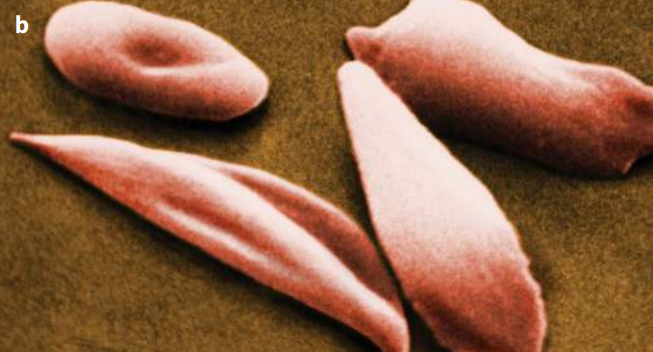
Each haem group contains an iron atom. One oxygen molecule, O2, can bind with each iron atom. So a complete hemoglobin molecule, with four haem groups, can carry four oxygen molecules (eight oxygen atoms) at a time. It is the haem group which is responsible for the color of hemoglobin. This color changes depending on whether or not the iron atoms are combined with oxygen.
If they are, the molecule is known as oxyhemoglobin, and is bright red. If not, the color is purplish.
Collagen – a fibrous protein
Collagen is the most common protein found in animals, making up 25% of the total protein in mammals. It is an insoluble fibrous protein (Figure M) found in skin (leather is preserved collagen), tendons, cartilage, bones, teeth and the walls of blood vessels. It is an important structural protein, not only in humans but in almost all animals, and is found in structures ranging from the body walls of sea anemones to the egg cases of dogfish.
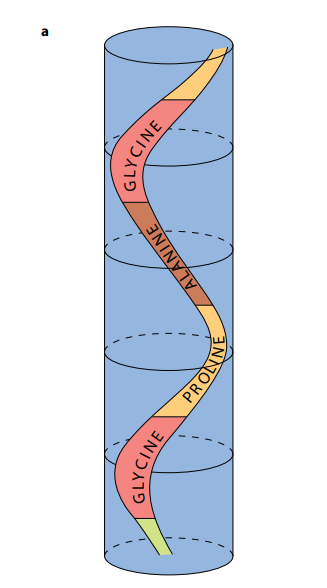
As shown in Figure (M2), a collagen molecule consists of three polypeptide chains, each in the shape of a helix. (This is not an α-helix – it is not as tightly wound.) These three helical polypeptides are wound around each other, forming a three-stranded ‘rope’ or ‘triple helix’. The three strands are held together by hydrogen bonds and some covalent bonds. Almost every third amino acid in each polypeptide is glycine, the smallest amino acid. Glycine is found on the insides of the strands and its small size allows the three strands to lie close together and so form a tight coil. Any other amino acid would be too large.
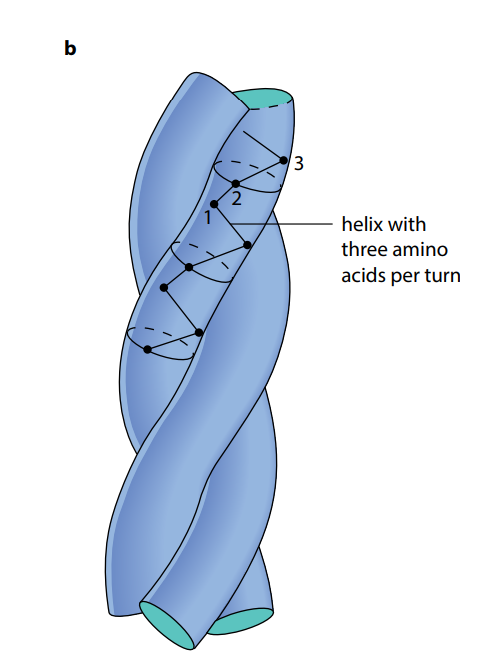
Each complete, three-stranded molecule of collagen interacts with other collagen molecules running parallel to it. Covalent bonds form between the R groups of amino acids lying next to each other. These cross-links hold many collagen molecules side by side, forming fibrils. The ends of the parallel molecules are staggered; if they were not, there would be a weak spot running right across the collagen fibril. Finally, many fibrils lie alongside each other, forming strong bundles called fibers.
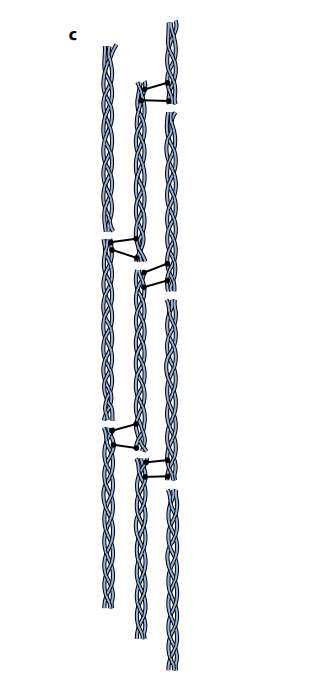
The advantage of collagen is that it is flexible but it has tremendous tensile strength, meaning it can withstand large pulling forces without stretching or breaking. The human Achilles tendon, which is almost pure collagen fibers, can withstand a pulling force of 300N per mm2 of cross-sectional area, about one-quarter the tensile strength of mild steel.
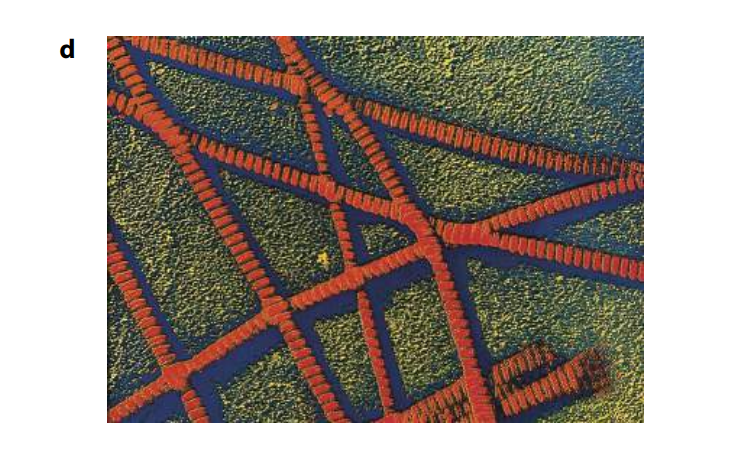
Collagen fibers line up according to the forces they must withstand. In tendons they line up in parallel bundles along the length of the tendon, the direction of tension. In skin, they may form layers, with the fibers running in different directions in the different layers, like cellulose in cell walls. In this way, they resist tensile (pulling) forces from many directions.
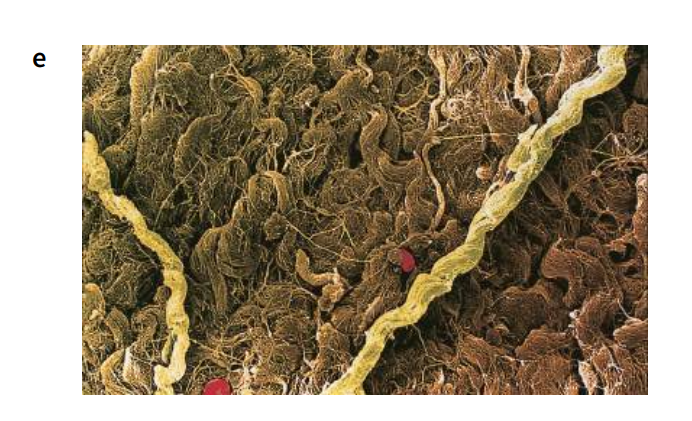
Proteins are long chains of amino acids which fold into precise shapes. Biuret reagent can be used to test for proteins. The linkages that join amino acids are called peptide bonds. The sequence of amino acids in a protein, known as its primary structure, determines the way that it folds and hence determines its three-dimensional shape and function.
Many proteins contain areas where the amino acid chain is twisted into an α-helix; this is an example of secondary structure. The structure forms as a result of hydrogen bonding between the amino acids. Another secondary structure formed by hydrogen bonding is the β-pleated sheet. Further folding of proteins produces the tertiary structure. Often, a protein is made from more than one polypeptide chain. The association between the different chains is the quaternary structure of the protein. Tertiary and quaternary structures are very precise and are held in place by hydrogen bonds, disulfide bonds (which are covalent), ionic bonds and hydrophobic interactions.
Proteins may be globular or fibrous. A molecule of a globular protein – for example hemoglobin – is roughly spherical. Most globular proteins are soluble and metabolically active. Hemoglobin contains a non-protein (prosthetic) group, the haem group, which contains iron. This combines with oxygen. A molecule of a fibrous protein – for example, collagen – is less folded and forms long strands. Fibrous proteins are insoluble. They often have a structural role. Collagen has high tensile strength and is the most common animal protein, being found in a wide range of tissues.
Reference
This Article is based on by the lecture of Mary Jones And Richerd Frosbery, Cambridge International University.
Some info and pictures have been added by Author and Wikipedia.
Reference used for info: Cambridge International Biology Course Book by Mary Jones.
Your article helped me a lot, is there any more related content? Thanks!