Proteins are large biomolecules, or macromolecules, consisting of one or more long chains of amino acid residues. Proteins perform a vast array of functions within organisms, including catalysing metabolic reactions, DNA replication, responding to stimuli, providing structure to cells, and organisms, and transporting molecules from one location to another. Proteins differ from one another primarily in their sequence of amino acids, which is dictated by the nucleotide sequence of their genes, and which usually results in protein folding into a specific 3D structure that determines its activity.
Proteins are an extremely important class of macromolecule in living organisms. More than 50% of the dry mass of most cells is protein. Proteins have many important functions. For example:
- all enzymes are proteins
- proteins are essential components of cell membranes – their functions in membranes, such as receptor proteins and signaling proteins.
- some hormones are proteins – for example, insulin and glucagon
- the oxygen-carrying pigments hemoglobin and myoglobin are proteins
- antibodies, which attack and destroy invading microorganisms, are proteins
- collagen, another protein, adds strength to many animal tissues, such as bone and the walls of arteries
- hair, nails and the surface layers of skin contain the protein keratin
- actin and myosin are the proteins responsible for muscle contraction
- proteins may be storage products – for example, casein in milk and ovalbumin in egg white.
Despite their tremendous range of functions, all proteins are made from the same basic monomers. These are amino acids.
Amino acids
Figure (A) shows the general structure of all amino acids and of glycine, the simplest amino acid. They all have a central carbon atom which is bonded to an amine group, –NH2, and a carboxylic acid group, –COOH. It is these two groups which give amino acids their name. The third component that is always bonded to the carbon atom is a hydrogen atom.
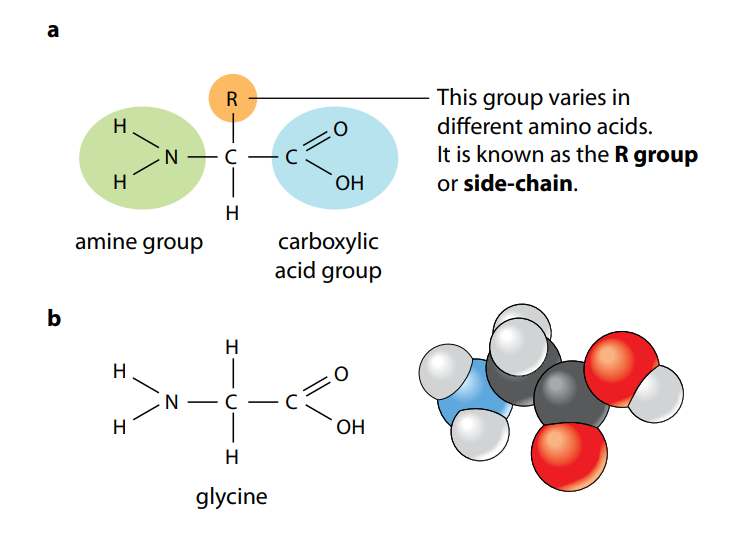
The only way in which amino acids differ from each other is in the remaining, fourth, group of atoms bonded to the central carbon. This is called the R group. There are 20 different amino acids which occur in the proteins of living organisms, all with a different R group. You can see their molecular formulae in Figure (B). (You do not need to remember all the different R groups.) Figure (B) also shows the three-letter abbreviations commonly used by scientists for the names of the amino acids. Many other amino acids have been synthesized in laboratories.
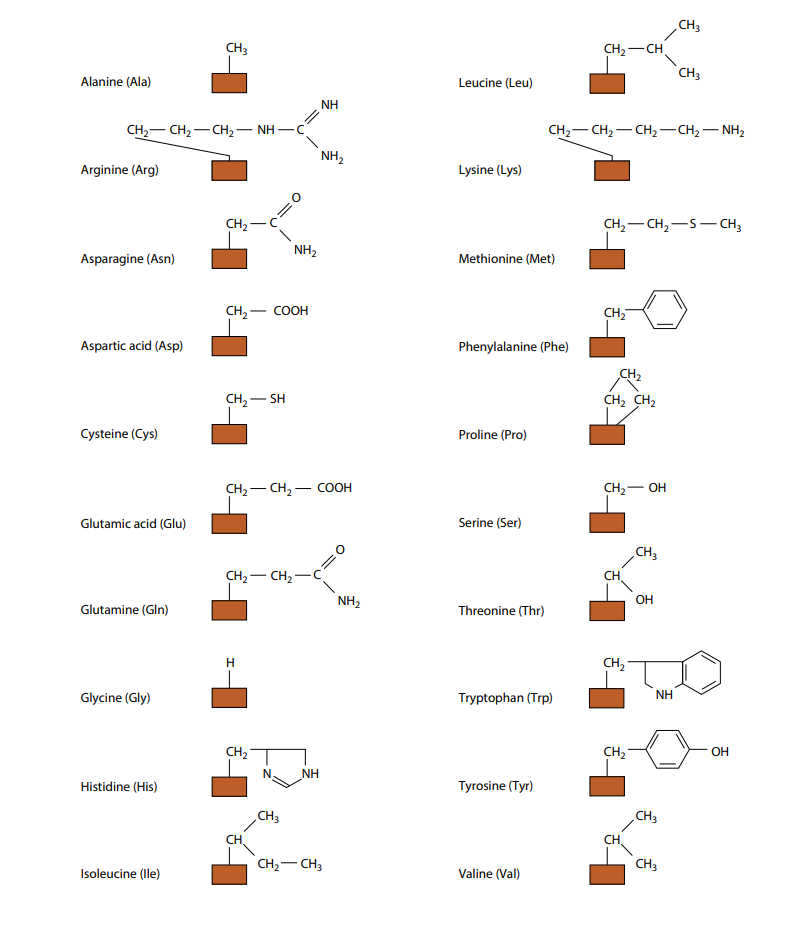
The peptide bond
Figure (C) shows how two amino acids can join together. One loses a hydroxyl (–OH) group from its carboxylic acid group, while the other loses a hydrogen atom from its amine group. This leaves a carbon atom of the first amino acid free to bond with the nitrogen atom of the second. The link is called a peptide bond. The oxygen and two hydrogen atoms removed from the amino acids form a water molecule. We have seen this type of reaction, a condensation reaction, in the formation of glyosidic bonds and in the synthesis of triglycerides.
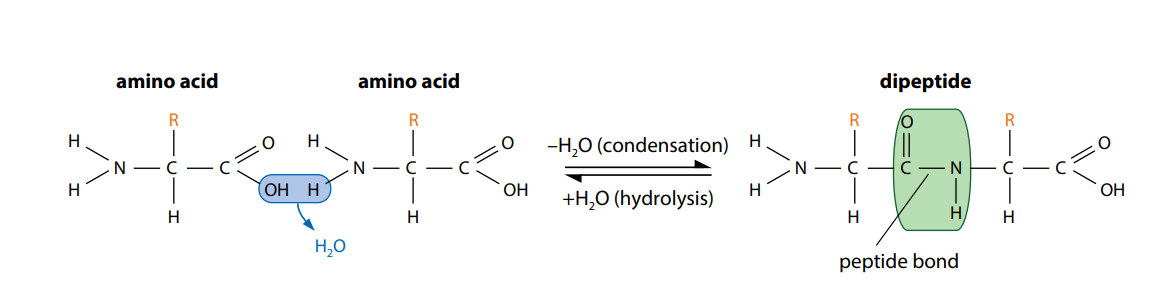
The new molecule which has been formed, made up of two linked amino acids, is called a dipeptide. Any number of extra amino acids could be added to the chain in a series of condensation reactions. A molecule made up of many amino acids linked together by peptide bonds is called a polypeptide. A polypeptide is another example of a polymer and a macromolecule, like a polysaccharide. A complete protein molecule may contain just one polypeptide chain, or it may have two or more chains which interact with each other.
In living cells, ribosomes are the sites where amino acids are joined together to form polypeptides. The reaction is controlled by enzymes.
Polypeptides can be broken down to amino acids by breaking the peptide bonds. This is a hydrolysis reaction, involving the addition of water (Figure C), and happens naturally in the stomach and small intestine during digestion. Here, protein molecules in food are hydrolyzed into amino acids before being absorbed into the blood.
Primary structure
A polypeptide or protein molecule may contain several hundred amino acids linked into a long chain. The particular amino acids contained in the chain, and the sequence in which they are joined, is called the primary structure of the protein. Figure (D) shows the primary structure of the protein ribonuclease, an enzyme. There are an enormous number of different possible primary structures. Even a change in one amino acid in a chain made up of thousands may completely alter the properties of the polypeptide or protein.
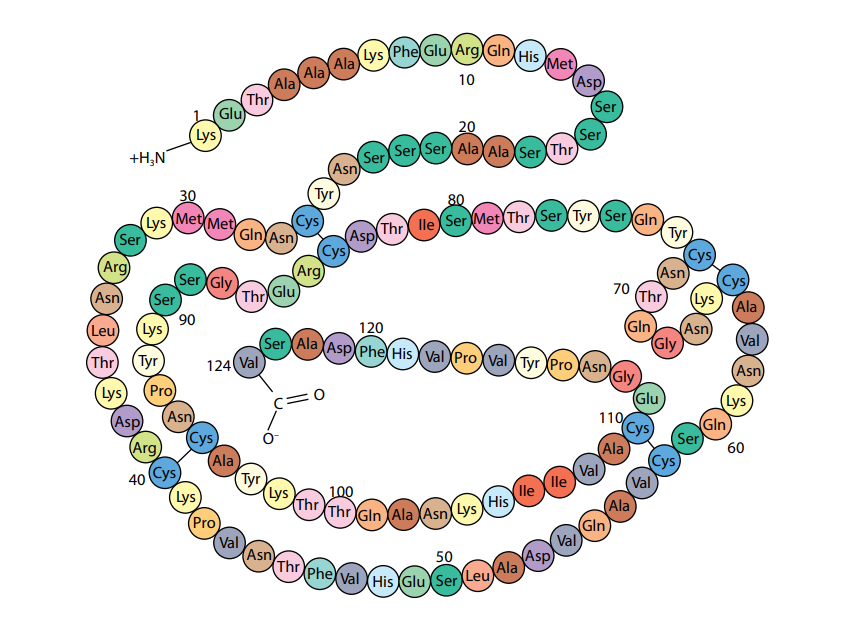
Secondary structure
The amino acids in a polypeptide chain have an effect on each other even if they are not directly next to each other. A polypeptide chain, or part of it, often coils into a corkscrew shape called an α-helix (Figure E1). This secondary structure is due to hydrogen bonding between the oxygen of the –CO– group of one amino acid and the hydrogen of the –NH– group of the amino acid four
places ahead of it. Each amino acid has an –NH– and a –CO– group, and Figure E1 shows that all these groups are involved in hydrogen bonding in the α-helix, holding the structure firmly in shape. Hydrogen bonding is a result of the polar characteristics of the –CO– and –NH– groups.
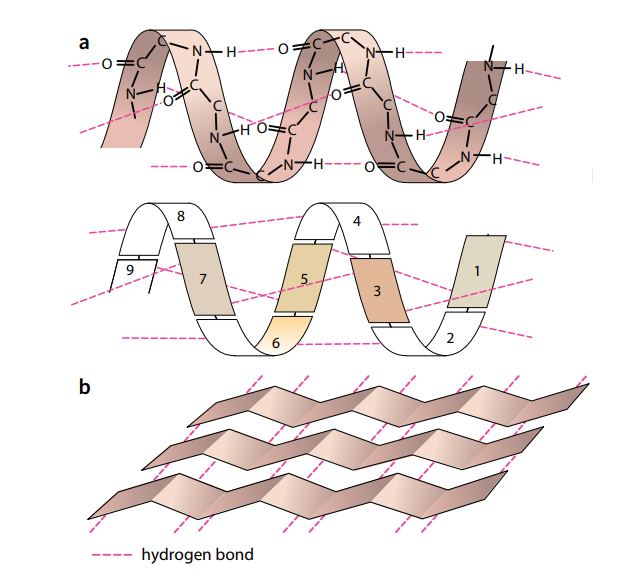
Sometimes hydrogen bonding can result in a much looser, straighter shape than the α-helix, which is called a β-pleated sheet (Figure E2). Hydrogen bonds, although strong enough to hold the α-helix and β-pleated sheet structures in shape, are easily broken by high temperatures and pH changes. As you will see, this has important consequences for living organisms.
Some proteins or parts of proteins show no regular arrangement at all. It all depends on which R groups are present and therefore what attractions occur between amino acids in the chain.
In diagrams of protein structure, α-helices can be represented as coils or cylinders, β-sheets as arrows, and random coils as ribbons (Figures F and Figure G).
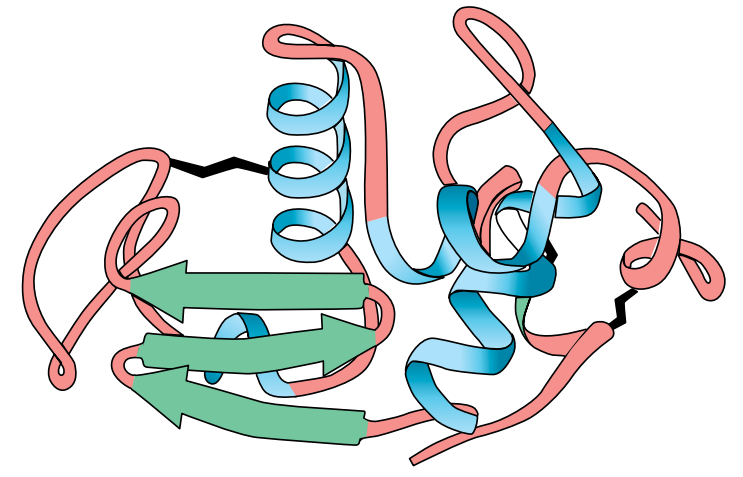
Tertiary structure
In many proteins, the secondary structure itself is coiled or folded. Figure (F) shows the complex way in which a molecule of the protein lysozyme folds. Here the α-helices are represented as coils, while in Figure (G), which shows the secondary and tertiary structure of myoglobin, the α-helices are shown as cylinders.
At first sight, the myoglobin and lysozyme molecules look like disorganized tangles, but this is not so. The shape of the molecules is very precise, and the molecules are held in these exact shapes by bonds between amino acids in different parts of the chain. The way in which a protein coils up to form a precise three-dimensional shape is known as its tertiary structure.
Primary structure is the sequence of amino acids in a polypeptide or protein.
Secondary structure is the structure of a protein molecule resulting from the regular coiling or folding of the chain of amino acids, e.g. an α-helix or β-pleated sheet.
Tertiary structure is the compact structure of a protein molecule resulting from the three-dimensional coiling of the already-folded chain of amino acids.
Quaternary structure is the three-dimensional arrangement of two or more polypeptides, or of a polypeptide and a non-protein component such as haem, in a protein molecule.
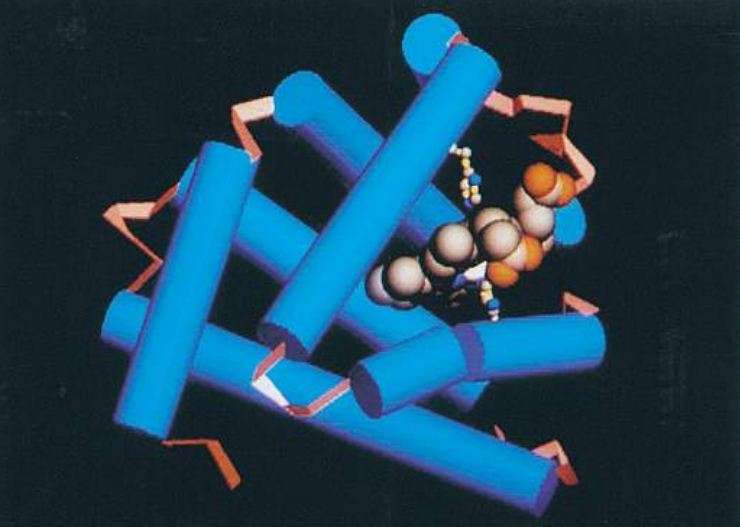
Figure (H) shows the four types of bond which help to keep folded proteins in their precise shapes. Hydrogen bonds can form between a wide variety of R groups. Disulfide bonds form between two cysteine molecules, which contain sulfur atoms. Ionic bonds form between R groups containing amine and carboxyl groups. Hydrophobic interactions occur between R groups which are non-polar, or hydrophobic.
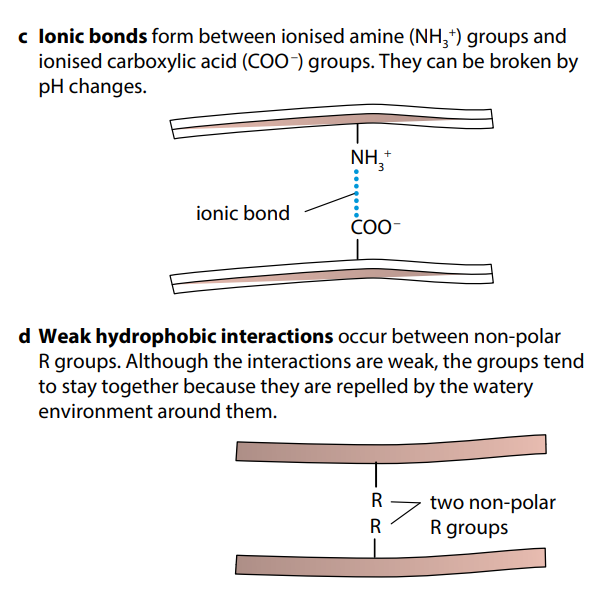
Quaternary structure
Many protein molecules are made up of two or more polypeptide chains. Hemoglobin is an example of this, having four polypeptide chains in each molecule (Figure I). Th e association of different polypeptide chains is called the quaternary structure of the protein. The chains are held together by the same four types of bond as in the tertiary structure. More details of hemoglobin are given in the next section.
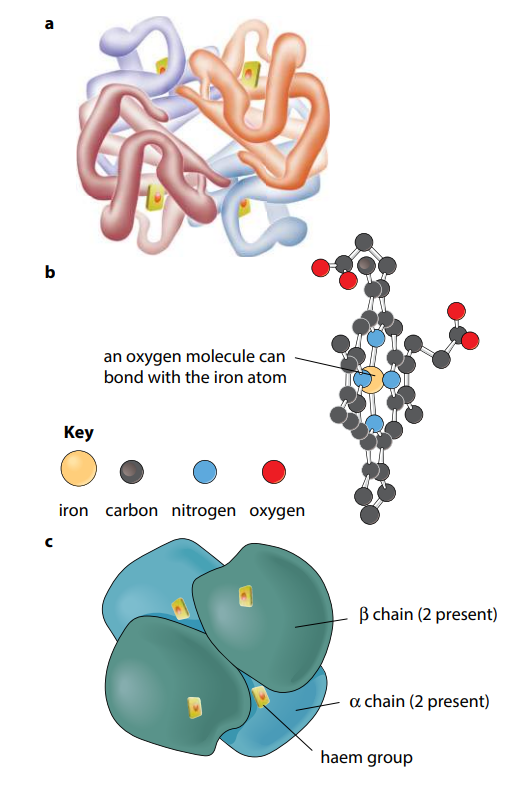
Chek out the other part of this article in Protein and Peptides: An Inevitable Source of Nutrition (Part 2).
Reference
This Article is based on by the lecture of Mary Jones And Richerd Frosbery, Cambridge International University.
Some info and pictures have been added by Author and Wikipedia.
Reference used for info: Cambridge International Biology Course Book by Mary Jones.